Abstract
Computer simulations of strongly-correlated quantum materials, such as high-temperature superconductors, are generally intractable with classical computational methods. Quantum computers and simulators offer a way forward by leveraging inherently quantum systems. Quantum simulation encodes quantum models in controllable atomic arrays, providing an analog approach to studying strongly-correlated quantum phenomena. By contrast, quantum computing offers a more general framework with the potential for exponential speedup across a wide range of problems but requires overcoming significant engineering challenges in scalability, optical control, and system integration. In this talk, I will present my work on engineering neutral atom quantum systems for both quantum simulation and computation.
During my PhD, I developed the first fermionic quantum gas microscope, enabling single-atom imaging of a strongly correlated quantum system. This platform provided the first direct observations of spin correlations and long-range antiferromagnetic order in the Hubbard model, which is predicted to contain the essential ingredients of high-temperature superconductivity. Building this quantum simulator required a number of engineering advances, including ultra-low-noise, high-power lasers, dynamically configurable optical traps, and a novel imaging technique that combined laser cooling with fluorescence imaging, all controlled by a custom-built FPGA-based system. Following my PhD, I spent six years in industry, where I designed Atom Computing’s first prototype neutral atom quantum computer and developed miniaturized, highly integrated, optoelectronic systems for augmented reality displays at Meta. I am now applying some of these engineering advances to develop a neutral atom quantum testbed focused on developing scalable optical control of neutral atom qubits. I will discuss our initial efforts toward utility-scale quantum computing by implementing three-dimensional arrays of qubits using advanced techniques in digital holographic projection.
Bio
The Parsons Lab focuses on advancing the frontiers of quantum computing and quantum sensing through innovative experimental techniques. Our research is centered on developing scalable quantum technologies, including three-dimensional arrays of neutral atoms for quantum simulation and computation, and leveraging novel nanophotonic components to enhance quantum systems. We are also exploring solid-state platforms, such as nitrogen-vacancy centers in diamond and other color centers, to build hybrid quantum architectures and spin-photon interfaces. Max also leads the Quantum Technologies Training and Testbed Lab (QT3), which serves as a shared user facility, an instructional laboratory, and quantum testbeds. The QT3 lab is staffed primarily by undergraduate researchers, emphasizing hands-on experiential learning and fostering the next generation of quantum scientists and engineers. Our work bridges fundamental physics, advanced engineering, and interdisciplinary collaboration to address some of the most challenging problems in quantum science and technology.
Max completed his PhD in 2016 under the supervision of Markus Greiner at Harvard University, where he developed techniques for laser cooling and atom-resolved imaging of fermionic lithium atoms in optical lattices for quantum simulation experiments. Prior to joining UW in 2022, Max worked as a scientist at Reality Labs (Meta) where he worked on augmented reality display systems, involving research on pupil replicating waveguides, laser beam scanning projectors, novel emitters, and digital holographic imaging. Max also worked as a scientist at Atom Computing, developing gate-based quantum computers based on arrays of neutral atoms trapped in optical tweezers. In addition to a number of academic publications, Max is an inventor on more than 35 granted patents in the fields of mixed reality and quantum computing.
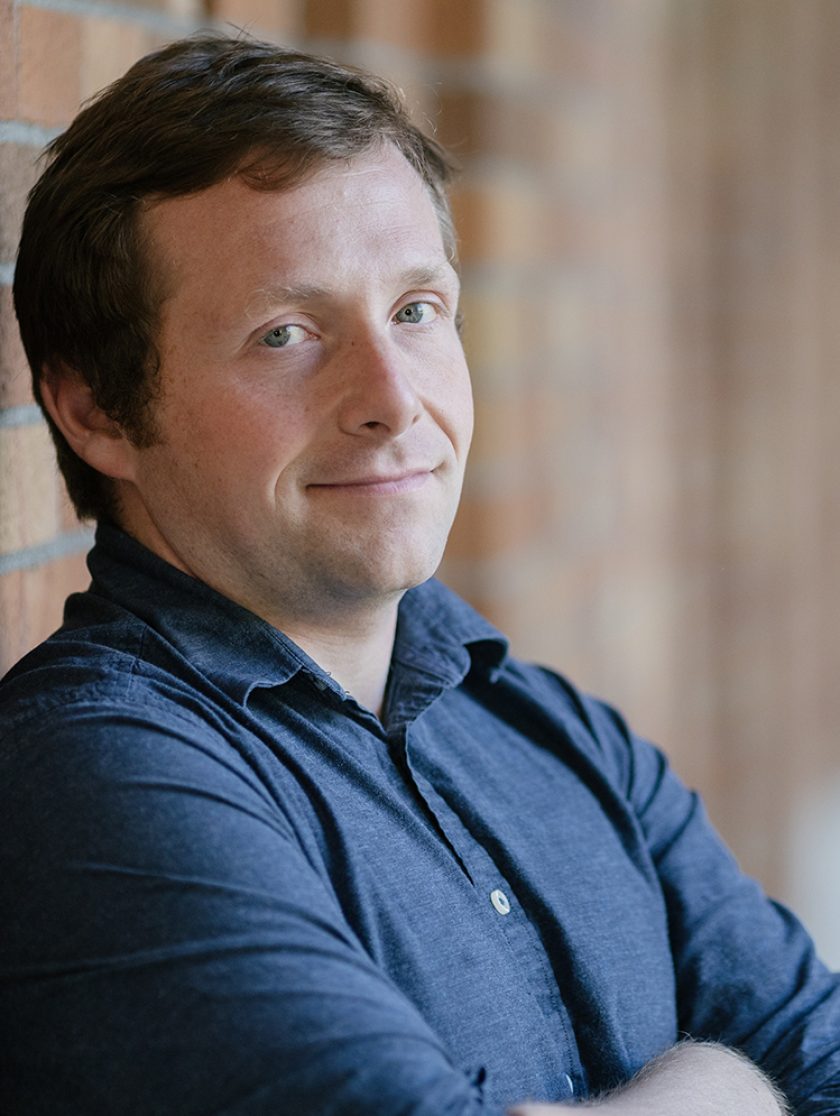